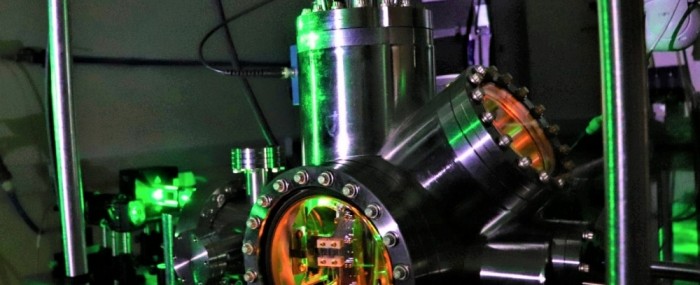
Research lays foundation for future development of ultraviolet LEDs
23 de novembro de 2021By José Tadeu Arantes | Agência FAPESP – As an electrical insulator that withstands high temperatures, boron nitride is a material with many commercial applications. New functions that can be explored include production of ultraviolet C light-emitting diodes (UVC LEDs).
This type of light can sterilize spaces, surfaces and water by disrupting the DNA of microorganisms and making them inactive. Fluorescent tubes are currently used as UVC sources, but LEDs can be much more efficient, as they have already proved when used to light offices and homes.
A study designed to enhance understanding and control of the electronic and optical properties of boron nitride so that these new applications can be developed was conducted at the University of Campinas’s Gleb Wataghin Institute of Physics (IFGW-UNICAMP) in the state of São Paulo, Brazil. An article reporting the study is published in the journal 2D Materials.
The principal investigator was Luiz Fernando Zagonel, a professor at IFGW-UNICAMP’s Photovoltaic Research Laboratory (Department of Applied Physics). The research was conducted by PhD candidate Ricardo Javier Peña Román, a student of Zagonel, with a scholarship from FAPESP.
The study involved collaboration with research groups in the United Kingdom and France and was supported by FAPESP (besides the doctoral scholarship) via a Young Investigator grant and a Multiuser Equipment grant for the purchase of a scanning tunneling microscope, the main piece of equipment used in the study.
“Simply put, what we did was measure the electronic band gap of a monolayer of hexagonal boron nitride [h-BN],” Zagonel told Agência FAPESP.
A number of terms should be explained to help the reader understand what this involves. The electronic band gap, a significant property in solid-state physics, is the difference between the valence band and conduction band of electrons. The valence band in electrical insulators and semiconductors is the highest range of electron energies in which electrons are normally present at absolute zero. The electrons present in the valence band are loosely bound to the nucleus of the atom. The conduction band, located in the outermost layer of the material, consists of fewer electrons (none at all in some conditions) that can occupy many available states. These electrons have great mobility and are responsible for electrical conductivity.
“The electronic band gap can also be interpreted as a gap in the material’s electron distribution, in which there can be no electrons. It’s also known as the ‘forbidden band’ because it corresponds to an energy band that no electrons in the material can have,” Zagonel explained.
The main advantage of boron nitride in terms of applications is that it has a very wide band gap. In other words, to move an electron from the valence band to the conduction band, very little energy needs to be injected into the material. When the electron returns to the valence band, which is a more stable configuration, most of this energy flows back in the form of high-frequency electromagnetic radiation, or UVC.
“We determined the value of the electronic band gap, which is 6.8 electron-volts [eV]. The energy emitted by the material in the form of electromagnetic radiation is 6.1 eV. The difference, 0.7 eV, corresponds to the exciton binding energy, whose calculation is another important result of our study,” Zagonel said.
Excitons are pairs comprising conduction band electrons and valence band holes (absence of electrons), which are mutually attracted and bind to form pairs that orbit each other. The associated energy is called exciton binding energy, and its magnitude indicates the stability of the electron-hole pair, i.e., how long it is expected to remain stable at a given temperature.
“When an exciton recombines, so that the electron migrates to the gap state and fills it, this recombination can cause light emission with a level of energy corresponding to the energy of the electronic band gap minus the exciton binding energy,” Zagonel said.
At room temperature, solids are subject to temperature-associated thermal vibrations that may destabilize excitons with low binding energy, breaking the bonds between electrons and holes. If the bonds are broken very easily, exciton emission becomes unlikely, and the material behaves as an inefficient light emitter. For the material to be suited to technological applications that can be used in everyday life, the level of exciton binding energy must be higher than the energy of the vibrations associated with room temperature.
“Ideally, a majority of the electrons located in the conduction band should recombine with holes and emit light excitonically even at room temperature. This highly efficient light emission even at room temperature is what we found in the case of boron nitride,” Zagonel said.
Point defects
As well as measuring the energy associated with the electronic band gap and exciton binding energy, the researchers also investigated point defects in the material. These are atomic-scale dimensionless defects consisting basically of points where atoms of boron nitride are replaced by carbon atom nuclei.
“Using several experimental techniques with our scanning tunneling microscope [STM], we were able to record images of point defects, determine their energy levels, and observe the light emitted by them,” Zagonel said. “We obtained images showing general aspects of surface morphology and observed distinct regions with and without point defects. In both cases, we measured the density of the local electronic states by scanning tunneling spectroscopy [STS]. The results enabled us to determine the size of the band gap in boron nitride monolayers, a question never before resolved in the literature.”
In addition, they used a detection system on the microscope to analyze light emission associated with point defects. They measured photoluminescence (PL) and cathodoluminescence (CL), observing a distinct emission line at 2.1 eV, typically associated with carbon atoms.
“It’s worth stressing that this is the first characterization by CL in boron nitride monolayers reported in the literature. The presence of other emissions at higher energy levels suggests the coexistence of more than one kind of carbon defect in the material,” Zagonel said.
The STM used by the researchers runs in an ultra-high vacuum at cryogenic temperatures as low as 12 Kelvin (-261 °C), and has an innovative light detection system developed, implemented and patented by the team.
“The results obtained demonstrate the versatility of our setup, which is a powerful tool for characterizing two-dimensional materials and surfaces,” Zagonel said. “Our ongoing research focuses on materials of interest for the development of applications in devices.”
The article “Band gap measurements of monolayer h-BN and insights into carbon-related point defects” is at: iopscience.iop.org/article/10.1088/2053-1583/ac0d9c.